Introduction: Understanding the Quadruped TFL-Equivalent
The Tensor Fasciae Latae (TFL) is a small yet crucial muscle in human anatomy, playing a significant role in stabilizing the pelvis, assisting in lower limb movement, and contributing to the complex mechanics of bipedal locomotion. However, to truly grasp the functional significance of the human TFL, it is essential to explore its roots in comparative anatomy. By studying the anatomy of quadrupeds, we can better understand the evolutionary adaptations that led to the development of the TFL as a specialized structure in humans. Comparative studies not only shed light on the evolutionary path from quadrupedal to bipedal locomotion but also help elucidate the broader biomechanical principles that govern movement across species.
In quadrupeds, muscles located in the lateral hip region—often considered TFL-equivalents—serve functions that parallel those of the human TFL, albeit with adaptations specific to their four-legged locomotion. These muscles stabilize the hips, assist in flexion and extension of the hind limb, and play a key role in maintaining balance during movement. The study of these TFL-like muscles in animals such as dogs, cats, and horses reveals how evolutionary pressures shaped the musculature and connective tissue to meet the demands of speed, endurance, and agility in quadrupedal locomotion. By comparing these adaptations to the human TFL, we can better understand how bipedalism emerged as a distinct and efficient form of movement in humans.
One fascinating aspect of the TFL is its connection to the iliotibial (IT) band, a dense band of fascia that runs along the lateral thigh. While this connection is prominent in humans, the concept of a fascial extension for force distribution and stability is also present in quadrupeds. For example, horses possess a superficial gluteal muscle with a fascial component resembling the IT band, demonstrating the evolutionary continuity of this structure. These anatomical parallels suggest that the human TFL may have evolved as an adaptation of similar musculature in quadrupeds, refined to support the unique demands of upright walking and running.
Another intriguing dimension of TFL research is the groundbreaking discovery made by Dr. Carolyn Eng, which highlights the TFL’s additional role in shock absorption. Eng found that the TFL has a direct fascial branch extending to the lateral meniscus of the knee, indicating that its function extends beyond pelvic stabilization and hip movement. This branch allows the TFL to assist in protecting the knee joint during high-impact activities, such as running or jumping, by distributing forces away from the meniscus. This finding challenges the traditional understanding of the TFL’s function, positioning it as a key player in preserving joint integrity and enhancing movement efficiency.
Dr. Eng’s discovery not only underscores the TFL’s complexity but also offers a unique lens through which to examine its evolutionary origins. The ability to absorb shock and protect the knee is particularly advantageous for bipedal locomotion, where the lower limbs bear the entirety of the body’s weight during each step. By studying TFL-like muscles in quadrupeds, researchers can identify whether similar shock absorption mechanisms exist in four-legged animals or whether this feature emerged as a distinct adaptation to bipedalism.
The study of the quadruped TFL-equivalent provides an invaluable framework for understanding the evolution of human biomechanics. By examining the anatomical features and functions of these muscles in animals, we can trace the incremental changes that led to the emergence of bipedal locomotion—a hallmark of human evolution. This exploration not only enriches our understanding of human anatomy but also broadens our appreciation for the interconnectedness of form and function across species.
In this discussion, we will delve into the attachments, functions, and evolutionary significance of the TFL and its quadruped equivalents. By integrating insights from comparative anatomy and groundbreaking research, such as Dr. Eng’s discovery, we aim to provide a comprehensive understanding of how this small but vital muscle evolved to meet the biomechanical challenges of bipedalism. Through this lens, the TFL emerges as not merely a stabilizer of the pelvis but as a dynamic, multifunctional structure that bridges the gap between evolutionary history and modern human movement.
The TFL or “TFL-like” Muscles in Animals
The Tensor Fasciae Latae (TFL) is a key muscle in human biomechanics, renowned for its role in stabilizing the pelvis during walking, running, and other forms of bipedal movement. Positioned on the lateral aspect of the thigh, it connects to the iliotibial (IT) band, which extends down to the tibia, providing additional support and assisting in the complex coordination of lower limb motion. This muscle is particularly important in humans because it helps maintain balance and pelvic alignment when standing or moving on two legs.
Interestingly, while humans are unique in their reliance on bipedal locomotion, many four-legged animals also possess a muscle—or a group of muscles—in a similar anatomical region, performing comparable biomechanical functions. These “TFL-like” muscles often assist in stabilizing the hips and facilitating limb movement, albeit in ways that cater to the distinct movement patterns of quadrupeds.
In quadrupeds, such as dogs, cats, and horses, there is typically a muscle near the front of the hip that attaches to a fascial band running down the thigh. While the specific name and structure of this muscle can vary depending on the species, its functional role often aligns closely with that of the human TFL. For instance, in horses, the superficial gluteal muscle connects to a fascial extension similar to the human IT band. Though this structure differs anatomically, it performs an equivalent function, contributing to the stability of the hind leg and assisting in coordinated hip flexion and limb propulsion.
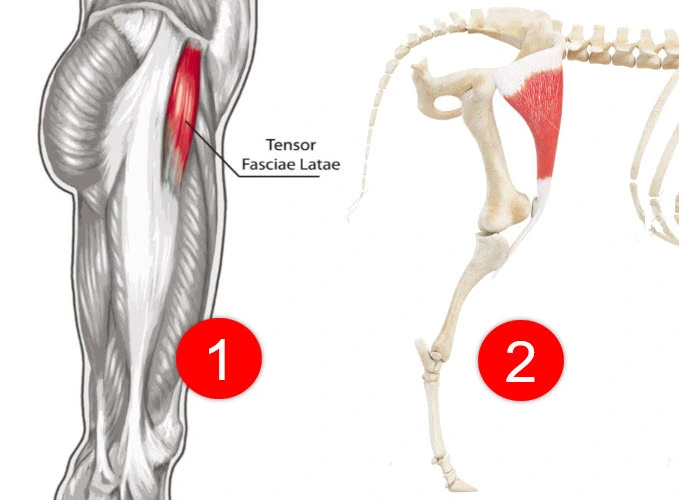
On the right, the horse’s superficial gluteal muscle is shown in red, reflecting a “TFL-like” role in quadrupedal animals. Though it differs anatomically from the human TFL, it performs comparable functions, such as stabilizing the hip and aiding hind limb propulsion. In horses, this muscle merges with a fascial sheet to support the stifle (knee) during high-speed gaits. The equine pelvis is elongated and oriented to distribute weight across four limbs, emphasizing powerful extension for galloping. By contrasting these two structures, we can observe how evolutionary pressures shape the interplay between muscle and fascia, optimizing locomotion for species-specific demands.
These adaptations reflect the evolutionary demands placed on each species. For animals like horses, which rely on swift and sustained locomotion, the TFL-like muscle and its associated fascial structures help absorb shock and distribute forces across the hip and thigh, optimizing efficiency during galloping or running. In smaller quadrupeds, such as cats, this muscle aids in the agility and flexibility required for rapid directional changes and jumping.
Understanding the similarities and differences in TFL-like muscles across species offers fascinating insights into the biomechanics of movement. It highlights how evolution has fine-tuned these structures to meet the demands of diverse locomotor strategies, from the upright gait of humans to the dynamic quadrupedal movements of other mammals.
Purpose in Quadrupeds
Before humans evolved to stand and move on two legs, the TFL-like muscles in quadrupedal ancestors played vital roles in maintaining stability and facilitating movement. These muscles and their associated fascial structures were critical for the mechanics of quadrupedal locomotion, contributing to various functions necessary for survival and efficiency.
1. Stabilizing the Hind Limb
In quadrupeds, these muscles were essential for keeping the hind limb aligned during weight-bearing activities. This function was particularly important during faster gaits like running or galloping, where the forces on the limbs are significantly increased. The tension provided by the muscle and fascia helped ensure that the limb maintained proper alignment, preventing energy loss and potential injury during high-speed movement.
2. Aiding Hip Flexion
Strong hip flexors are crucial for efficient locomotion in four-legged animals. TFL-like muscles in quadrupeds contributed to propelling the limb forward during each step cycle, working in concert with other muscles to generate the force required for movement. This function was vital not only for walking but also for rapid movements such as sprinting or leaping, where powerful hip flexion ensures swift and precise limb positioning.
3. Assisting Lateral Stability
Lateral stability is another critical function of TFL-like muscles in quadrupeds. The tension exerted through the associated fascial bands helped prevent the hip from swaying or collapsing outward during movement. Unlike humans, where this function is focused on stabilizing the pelvis during a single-leg stance, in quadrupeds, it supports the hips during alternating phases of locomotion. This ensures that the animal maintains balance and coordination, even during complex or rapid movements.
These roles underscore the evolutionary versatility of these muscles and their fascial connections. In quadrupeds, they worked as part of an integrated system to optimize locomotor efficiency and stability. By fulfilling these crucial functions, TFL-like muscles helped quadrupeds adapt to various environments and movement demands, laying the foundation for later adaptations seen in bipedal species.
The evolution of these muscles into the highly specialized TFL in humans highlights how anatomical structures are shaped by functional needs. In quadrupeds, their purpose centered on stabilizing, propelling, and balancing the body during four-legged movement. These roles were adapted and refined as our ancestors transitioned to upright locomotion, transforming these muscles into a key component of human biomechanics. Understanding the purpose of TFL-like muscles in quadrupeds allows us to appreciate their significance in the broader context of evolution and comparative anatomy.
Attachment in Quadrupeds: No Direct Meniscal Branch
The anatomy of the Tensor Fasciae Latae (TFL) or its equivalent in quadrupeds reflects its evolutionary adaptations for four-legged locomotion. Unlike in humans, where the TFL plays a dual role in stabilizing the pelvis and aiding knee function, most quadrupeds lack a direct attachment or “branch” from the TFL-like muscle to the lateral meniscus of the knee (known as the “stifle” in veterinary contexts). While there are variations among species such as horses, dogs, and cats, the general anatomical and functional characteristics of the TFL-like muscles in quadrupeds highlight their unique purpose in supporting movement.
Attachment Regions
The attachments of TFL or analogous muscles in quadrupeds are tailored to their locomotor needs:
- Origin: Typically, these muscles originate near the iliac crest or the tuber coxae, which are prominent features of the pelvic bone.
- Fascial Insertion: After originating from the pelvis, the muscle blends with the fascia lata, a broad sheet of connective tissue that envelops the thigh.
- Distal Insertions: The fascial extension of the TFL-like muscle often inserts in areas around the patella, the lateral patellar ligament, or the cranial aspect of the tibia.
These attachment points are designed to support the forces generated during quadrupedal locomotion, ensuring stability and efficiency in the hind limb’s movement.
No Direct Meniscal Attachment
Unlike the discovery in humans by Dr. Carolyn Eng, which revealed a direct fascial branch of the TFL extending to the lateral meniscus, this feature is absent in quadrupeds. The menisci in the stifle joint are fibrocartilaginous structures that primarily function as shock absorbers, and direct muscle or tendon attachments to these structures are rare or minimal across most species.
In quadrupeds:
- The TFL’s fascial extensions are closely associated with the lateral side of the stifle, but they do not directly insert onto the lateral meniscus.
- Other soft tissues, such as the joint capsule and specific ligaments, provide stability and anchor the menisci, but the TFL itself is not directly involved.
Functional Role of TFL in Quadrupeds
The absence of a direct meniscal attachment in quadrupeds does not diminish the importance of the TFL or its equivalent muscles. Instead, their functions are optimized for the demands of quadrupedal locomotion:
- Hip Stabilization and Support
The TFL-like muscle in quadrupeds works to stabilize the hip joint during weight-bearing. By tensing the fascia lata, it ensures the proper alignment of the hind limb, which is essential for maintaining stability during locomotion. - Hip Flexion
As a mild hip flexor, the TFL assists in lifting the hind limb during the swing phase of gait. This action is crucial for forward propulsion, especially during faster gaits such as running or galloping. - Stifle Support
Although it does not directly attach to the lateral meniscus, the TFL helps support the stifle joint by maintaining the tension of the fascia lata. This contributes to the overall stability of the knee and prevents excessive lateral or medial movement during locomotion. - Force Distribution
The TFL and its fascial connections distribute forces across the limb, reducing strain on individual muscles and joints. This function is particularly important during high-impact activities or when navigating uneven terrain.
Comparing to Humans: Evolutionary Insights
The absence of a direct meniscal branch in quadrupeds highlights a key distinction in the evolutionary trajectory of the TFL. In humans, the discovery of this branch undersc
Evolution Toward Bipedalism
The transition from quadrupedal locomotion to bipedal walking represents one of the most significant milestones in human evolution. This shift fundamentally altered the mechanics of movement and placed new demands on the musculoskeletal system, including the Tensor Fasciae Latae (TFL) muscle. As early human ancestors adapted to upright walking, the TFL evolved from primarily aiding in limb propulsion and hip stabilization in quadrupeds to playing a critical role in maintaining balance, stability, and efficiency during bipedal locomotion.
Pelvic Stabilization Became More Critical
One of the most significant changes during the evolution of bipedalism was the increased reliance on pelvic stabilization. Unlike quadrupeds, which distribute their body weight across four limbs, bipedal walking requires the pelvis to remain level and stable during the single-leg stance phase of gait. Without proper stabilization, the trunk would tilt excessively, leading to inefficient and unbalanced movement.
The TFL became a key player in addressing this challenge. Working in concert with the gluteus medius and gluteus minimus, the TFL helps prevent the pelvis from dropping on the side opposite to the supporting leg—a phenomenon known as the Trendelenburg sign when compromised. This adaptation allowed early bipedal walkers to move efficiently and maintain their center of gravity over the supporting limb, which is crucial for balance and forward propulsion.
Shift in Center of Gravity
The transition to bipedalism also necessitated a significant shift in the body’s center of gravity. In quadrupeds, the center of gravity is distributed along the length of the body, supported by four limbs. In bipedal humans, the center of gravity is located closer to the pelvis, directly above the supporting leg during walking.
This shift placed greater demands on the muscles responsible for hip abduction, rotational stability, and lateral balance. The TFL evolved to play a more prominent role in these functions. By working through its connection to the iliotibial (IT) band, the TFL helps stabilize the hip laterally, resist excessive medial or lateral rotation, and ensure smooth transitions between steps. This enhanced role was critical in adapting to the narrower base of support inherent in bipedal walking.
Changes in Muscle Function
In quadrupeds, TFL-like muscles primarily assist with hip flexion, stabilize the hind limb, and contribute to forward propulsion during movement. These functions were essential for efficient four-legged locomotion, where the limbs work in coordinated pairs to generate speed and stability.
As early humans evolved into upright walkers, the primary tasks of the TFL shifted:
- Single-Leg Support: In bipedal walking, there is a constant alternation between single-leg support and limb swing. The TFL became crucial in maintaining stability during the stance phase, preventing the body from tilting sideways.
- Force Distribution: The TFL’s connection to the IT band allows it to distribute forces along the lateral aspect of the thigh, reducing strain on the hip and knee joints during weight-bearing activities.
- Pelvic Rotation and Alignment: Bipedal walking involves a degree of pelvic rotation to facilitate stride length and efficiency. The TFL aids in maintaining proper alignment of the pelvis and lower limb, contributing to smooth and coordinated movement.
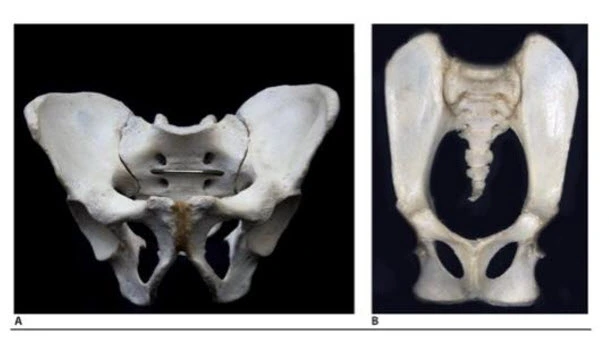
The images illustrate the structural differences between the pelvis of a quadruped (A) and a biped (B), highlighting how evolutionary adaptations reflect their respective modes of locomotion.
In quadrupeds (A), the pelvis is elongated and narrower, with a horizontal orientation that supports the distribution of weight across four limbs. This design is optimized for stability during quadrupedal movement, where the limbs work in coordinated pairs. The attachment points for muscles such as the gluteals and TFL-like muscles reflect the need for efficient limb propulsion and hip stabilization, enabling quadrupeds to excel in activities such as running and galloping.
In contrast, the biped pelvis (B) is shorter and wider, reflecting adaptations for upright locomotion. The broader structure shifts the center of gravity over the lower limbs, allowing for balance during single-leg support phases of walking or running. This design also supports critical functions such as pelvic stabilization, provided by muscles like the TFL and gluteus medius, which prevent lateral tilting of the trunk. The changes in shape also accommodate the biomechanical demands of childbirth in humans, further showcasing the functional versatility of the bipedal pelvis.
This comparison emphasizes how form and function are intertwined in the evolution of locomotor strategies.
Structural Adaptations
Structural Adaptations and the TFL’s Evolving Role
The evolution of bipedalism necessitated profound modifications to the human skeleton, particularly around the pelvis and lower limbs. As hominins began standing and walking on two legs, the pelvis underwent a transformation: it became shorter and broader, enhancing the mechanical advantage of several hip musculature components—most notably, the hip abductors such as the gluteus medius, gluteus minimus, and tensor fascia lata (TFL). A wider pelvis increases the moment arm for these muscles, allowing them to generate more force with less effort, a crucial adaptation for supporting the body’s weight on a single limb during each step of bipedal locomotion.
Additionally, the rearrangement of the femur contributed significantly to the emerging efficiency of human gait. The femur developed a pronounced valgus angle, bringing the knees in closer alignment beneath the body’s center of gravity. This shift was pivotal for balance and stability. Aligning the lower limbs more directly under the torso minimized side-to-side swaying, thereby reducing energy expenditure. It also enabled the TFL to collaborate more effectively with the other hip abductors in maintaining pelvic stability—particularly important during the mid-stance phase of gait, when the entire body weight is supported by one leg.
Another crucial adaptation involves the structural interplay between the TFL and the iliotibial band (ITB). The TFL’s insertion into the ITB means that when the TFL contracts, it tightens this broad connective tissue, providing lateral stabilization for the thigh and knee. In the context of a more valgus femur, this tension helps reduce medial or lateral shifts in knee alignment, protecting the joint from excessive stress and torsion. By curbing unsteady movements, the TFL plays a vital role in the spring-like mechanism of bipedal gait—storing and releasing energy as we step, further increasing locomotor efficiency.
Taken together, these adaptations underscore the TFL’s critical function in our unique form of upright movement. The structural changes in the pelvis—shaping it into a robust, bowl-like configuration—and the angling of the femur under the torso set the foundation for a more stable, energy-efficient stride. The TFL capitalizes on this arrangement by contributing both to hip abduction and lateral stabilization, embodying the sophisticated interplay between skeletal geometry and muscular function that distinguishes human bipedalism.
Energy Efficiency and Endurance
Bipedalism brought remarkable energy efficiency advantages over quadrupedal locomotion, particularly for long-distance travel, which was a key factor in the survival and success of early humans. By adopting an upright gait, early humans reduced the energy cost associated with maintaining stability and propulsion, enabling them to cover vast distances with less effort compared to their quadrupedal counterparts.
One critical factor in this efficiency was the role of the Tensor Fasciae Latae (TFL). As part of the lateral stabilizing system, the TFL helped maintain pelvic alignment and reduced unnecessary lateral or rotational movement during walking or running. These stabilizing actions were crucial in minimizing wasted energy that could otherwise occur from excessive swaying or imbalance. By keeping the body aligned and balanced during locomotion, the TFL allowed early humans to conserve energy, which could then be redirected toward essential survival tasks such as hunting, foraging, and evading predators.
Endurance walking and running—both hallmarks of human bipedalism—placed specific demands on the musculoskeletal system, particularly in terms of shock absorption and force distribution. The TFL, with its connection to the iliotibial (IT) band, played a significant role in distributing forces along the lateral aspect of the thigh, reducing strain on the hip and knee joints. This redistribution of forces not only protected these joints from injury but also enhanced overall efficiency by ensuring that the energy generated during movement was used effectively for forward propulsion rather than being dissipated through unnecessary movements.
The endurance capabilities provided by bipedalism were particularly important for early humans who engaged in persistence hunting—a hunting strategy where prey is pursued over long distances until it is exhausted. This hunting method required sustained locomotion over challenging terrains, often under the intense heat of the day. The TFL’s role in maintaining balance and reducing energy expenditure was vital in enabling early humans to excel in this form of endurance activity.
Furthermore, the upright posture associated with bipedalism freed the upper limbs for carrying tools, food, or offspring, adding to the overall survival advantage. The energy saved through the efficient stabilization provided by the TFL indirectly supported these additional tasks, enabling early humans to engage in complex behaviors that required both endurance and multitasking.
In modern humans, the energy-efficient mechanisms developed during the evolution of bipedalism remain essential. Activities such as long-distance running and walking continue to rely on the TFL for pelvic stabilization and efficient movement. However, contemporary lifestyles, which often involve prolonged periods of sitting or limited physical activity, can lead to imbalances or dysfunctions in the TFL, potentially diminishing the efficiency of these movements and causing discomfort or injury.
By understanding the role of the TFL in promoting energy efficiency and endurance during the evolution of bipedalism, we gain valuable insights into its critical contributions to human locomotion and the unique adaptations that have allowed our species to thrive in diverse environments. This perspective underscores the importance of maintaining the health and functionality of the TFL in supporting our ability to move efficiently and effectively, whether in daily activities or athletic pursuits.
A Revealing Study on its Elastic Role in Human Locomotion
Research by Carolyn Eng and colleagues sheds light on the intriguing mystery of the iliotibial band (ITB) fascia and its role in human locomotion. The ITB fascia, a large piece of elastic connective tissue that runs along the outer thigh, has long been associated with injury and pain in many people. However, Eng’s studies challenge the conventional wisdom that the IT fascia functions primarily to stabilize the hip during walking. Instead, they suggest that the IT fascia acts as an elastic structure that stores and releases energy to improve walking and running efficiency.
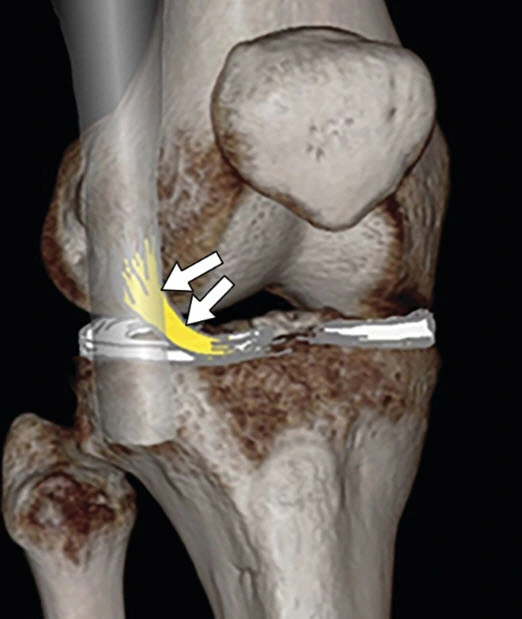
By connecting to the lateral meniscus, the ITB’s forces can be partially transferred to the meniscus, helping distribute load away from purely articular surfaces. This mechanism may aid in shock absorption during weight-bearing activities such as walking, running, or jumping. In essence, the ITB does not only function as a “check rein” against excessive knee varus or valgus movement; it also assists in fine-tuning rotational and compressive forces around the joint.
Although not extensively detailed in older anatomical texts, this ITB–meniscal attachment is garnering increased attention in research on knee biomechanics and injury prevention. Its presence can have clinical implications: excessive tension or abnormal mechanics in the ITB might place additional stress on the lateral meniscus, potentially contributing to lateral knee pain or degeneration over time. Conversely, proper conditioning and balanced muscular support can help optimize the ITB’s role, potentially protecting the meniscus against undue strain. Ultimately, this accessory ligament connection highlights the knee’s complexity and the importance of a holistic view when assessing lateral knee function or pathology.
Research has revealed that the human iliotibial band fascia has a remarkable capacity to store 15 to 20 times more elastic energy per body mass than its less developed structure in chimpanzees. This increased energy storage capacity is particularly pronounced during running, a more bouncy gait compared to walking. The researchers suggest that the role of the IT fascia in locomotion, particularly running, may involve recycling stored elastic energy, thereby contributing to energy savings during movement.
Carolyn Eng and colleagues have conducted extensive research on the iliotibial band (ITB) and its role in human locomotion. Their studies suggest that the ITB acts as an elastic structure that stores and releases energy to improve the efficiency of walking and running, challenging the conventional wisdom that it primarily serves to stabilize the hip.
One of the key articles on this topic is titled “ The human iliotibial band is specialized for elastic energy storage compared with the chimp fascia lata“, published in 2015 in the Journal of Experimental Biology . In this study, Eng and colleagues compared the ability of the human ITB to store elastic energy compared to the chimpanzee fascia lata, demonstrating that the human ITB is specialized for elastic energy storage, which contributes to the economy of bipedal locomotion.
Another relevant article is “ The capacity of the human iliotibial band to store elastic energy during running“, published in 2015 in the Journal of Biomechanics . This study evaluated the ability of the BIT to store and release elastic energy during running, using a musculoskeletal model to estimate BIT forces and deformations during running at different speeds.
For a more accessible presentation of this research, the article ” Understanding the IT band » published by the Harvard Gazette in 2015 provides a summary of Carolyn Eng’s findings on the function of the BIT in human locomotion.
These studies provide valuable insights into the function of the iliotibial band, highlighting its essential role in improving the energy efficiency of walking and running in humans.
Unlike traditional views that focus on the role of this fascia in stabilizing the hip during walking, the researchers approached their study from an evolutionary perspective. They compared this fascia in humans to its counterpart in chimpanzees, emphasizing the importance of considering adaptations for both walking and running in human evolution.
To understand the function of the IT fascia, the researchers developed a computational model to estimate its stretch and energy storage during walking and running. The model was based on measurements obtained by manipulating cadaveric limbs through various movements. The findings have implications for both basic science and clinical studies, potentially influencing athletic training programs and gait rehabilitation.
In the future, the researchers plan to expand their investigation to include other primate species adapted to running. Additionally, they plan to explore how a better understanding of the IT fascia could help prevent or treat injuries, particularly IT fascia syndrome, a common overuse injury in runners and cyclists. Using the computational models developed in this study, the researchers aim to estimate the force transmitted through the IT fascia in individuals with and without IT fascia pain, thereby providing a scientific basis for treating athletes with such injuries.
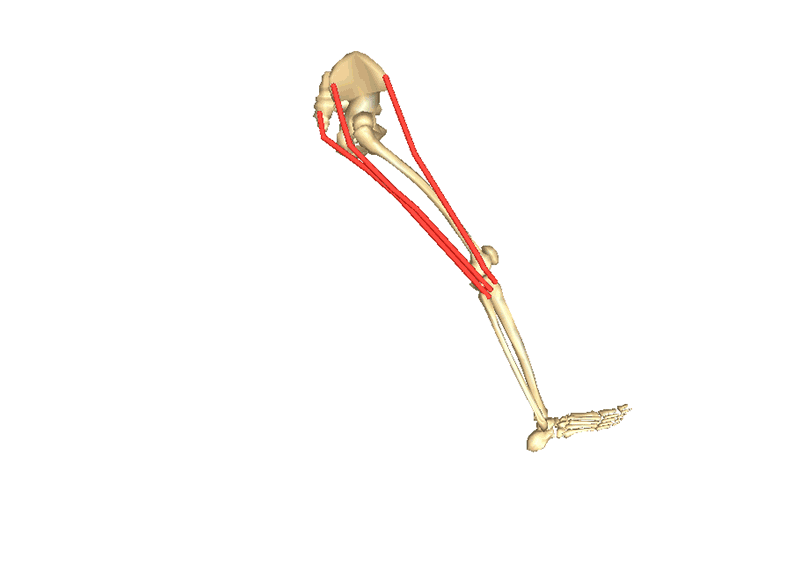
To uncover the role of the iliotibial (IT) band in human movement, researchers, led by Carolyn Eng, developed a sophisticated computer model to estimate how much the IT band stretches during walking and running — and consequently, how much energy it stores. The findings revealed that one section of the IT band stretches as the leg swings backward, storing elastic energy, which is then released as the leg moves forward, potentially reducing the energy cost of locomotion.
“It’s like recycling energy,” Eng explained. “Using these passive rubber bands instead of relying entirely on muscle activity makes movement more economical. The IT band stands out among human anatomical features as a potential key to improving the efficiency of running and even walking.”
Building the model was no simple task, as detailed descriptions of the IT band’s connections to muscles and bones were limited. To address this, Eng and her team turned to cadaver studies. Using a custom-built frame, they manipulated the hip and knee joints of five human and five chimpanzee cadaver limbs, measuring the changes in IT band length relative to joint angles.
These measurements helped calculate the IT band’s moment arm — the position relative to the hip and knee joints — which determines how much the IT band stretches and the torque it transmits during locomotion. The data provided insights into the IT band’s role in elastic energy storage, contributing to a more economical stride, and underscoring its importance as an adaptation for bipedal locomotion.
Credit: Carolyn Eng
Reference: The studies, co-authored with Daniel Lieberman, the Edwin M. Lerner II Professor of Biological Sciences and chair of the Department of Human Evolutionary Biology; Andrew Biewener, the Charles P. Lyman Professor of Biology; and Allison Arnold-Rife, a research associate in Biewener’s lab, are described in new articles in the Journal of Experimental Biology and the Journal of Biomechanics.
Implications for Modern Human Movement
The evolutionary history of the tensor fascia lata (TFL) provides valuable insights into its current functions—and occasional dysfunctions—in modern humans. As bipedal locomotion refined over millennia, the TFL emerged as an essential stabilizer, working in tandem with the gluteus medius and minimus to prevent excessive pelvic drop and maintain balanced, energy-efficient movement. This evolutionary legacy remains evident in our daily activities: whenever the body transitions onto a single supporting leg—during walking, running, climbing stairs, or simply balancing on one foot—the TFL comes into play.
Pelvic Instability: Overuse injuries and muscle fatigue can reduce the TFL’s effectiveness in stabilizing the pelvis, potentially causing compensatory issues up and down the kinetic chain (e.g., low back pain or foot/ankle problems).
Single-Leg Stability and Hip Control
In present-day humans, the TFL continues to be critical for single-leg support. It contracts to keep the pelvis level, preventing the torso from tilting away from the stance leg. This stability is most apparent during the mid-stance phase of walking or running, when the contralateral (non-weight-bearing) foot is off the ground. Without an adequately functioning TFL (and the broader abductor group), the body’s center of gravity would shift excessively from side to side, leading to an inefficient gait and potentially contributing to injury.
Integration with the Iliotibial Band (ITB)
Much like our hominin ancestors, modern humans rely on the TFL’s ability to tension the ITB—a broad, fibrous band running along the lateral thigh. When the TFL tightens the ITB, it helps stabilize the knee laterally, absorbing and redistributing forces transmitted from the ground. This mechanism is especially important during high-impact activities such as running and jumping, where the ITB’s tension can mitigate stress on the knee joint. Evolutionary changes to the pelvis and lower limbs synergize with the TFL/ITB complex to produce a more efficient and durable gait cycle.
Demands of Contemporary Lifestyles
Despite its evolved efficiency, the TFL faces challenges in our modern world. Prolonged sitting—a staple of office-bound lifestyles—places the hips in constant flexion, potentially causing the TFL to remain in a semi-contracted state. Over time, this can lead to tightness or overactivity of the TFL, potentially compromising the balance between the TFL and other hip stabilizers (such as the gluteus medius and minimus). Repetitive movements, like running or cycling, can further strain the TFL if the body’s biomechanics are compromised (e.g., from poor posture, weak core musculature, or inadequate hip mobility).
Preventing and Addressing TFL-Related Dysfunctions
Stretching and Mobility Work: Targeted stretches aimed at the lateral hip, including the TFL and ITB, can mitigate muscle imbalances. Myofascial release techniques—using foam rollers or massage—may also reduce tension.
Strengthening Synergists: Strengthening exercises for the gluteus medius, gluteus minimus, and core can help redistribute the workload appropriately, decreasing the risk of TFL overuse.
Postural and Gait Training: Poor posture or faulty running mechanics can exacerbate TFL strain. Correcting these patterns—through professional assessment, gait retraining, or posture-focused exercises—can alleviate chronic stress on the muscle.
Balanced Exercise Regimens: Cross-training strategies that incorporate both flexibility and strength exercises help maintain overall hip and lower limb health. Avoiding overly repetitive movement patterns also supports TFL integrity.
Common TFL-Related Issues
IT Band Syndrome: An overactive or tight TFL can contribute to increased tension in the IT band, exacerbating friction at the lateral knee.
Lateral Knee Pain: Beyond IT band syndrome, TFL dysfunction can create imbalance around the knee joint, leading to discomfort or instability.
Conclusion / Closing Thoughts
This comprehensive exploration of the quadruped TFL-equivalent and its evolutionary transformation into the specialized Tensor Fasciae Latae in humans illuminates how deeply interwoven structure and function are in the realm of locomotion. By comparing the anatomy and roles of TFL-like muscles in four-legged animals to our own bipedal adaptations, we gain a clearer perspective on the incremental anatomical shifts that paved the way for upright walking. In quadrupeds, these muscles primarily stabilize and propel the hind limbs, ensuring efficient movement over varying terrains. Yet, as hominin ancestors transitioned to bipedalism, the demands of single-leg support, pelvic stability, and shock absorption reshaped the TFL’s purpose and attachments.
One of the most striking findings reaffirmed through this discussion is Dr. Carolyn Eng’s discovery of the TFL’s fascial branch extending to the lateral meniscus in humans—an adaptation that underscores the TFL’s multifaceted role. Beyond merely keeping the pelvis level and assisting in hip abduction, the TFL also contributes to knee joint stability and resilience. This meniscal attachment highlights a sophisticated mechanism of force distribution, showing that a once “modest” muscle is, in fact, instrumental for preserving joint integrity under the load of bipedal gait. Its significance extends into clinical practice, where understanding this anatomical nuance can help healthcare professionals refine approaches to treating IT band syndrome, lateral knee pain, and other conditions tied to TFL dysfunction.
From an evolutionary biology standpoint, the TFL’s story is a testament to how selective pressures—such as the need for more efficient energy expenditure over long distances—drive morphological change. Early humans who could better stabilize their hips, minimize wasted motion, and absorb repeated ground forces had a competitive edge in hunting, foraging, and migrating. Today, the legacy of these adaptations persists in everything from our posture at a work desk to our stride during a marathon. Recognizing the TFL’s evolutionary heritage can thus inform not only our approach to physical training and rehabilitation but also our understanding of the fundamental mechanics that allow humans to thrive in a variety of environments.
Clinically, this evolutionary lens reminds us that the TFL is not simply another muscle to be stretched or strengthened in isolation. Rather, it is a dynamic component of a complex musculoskeletal network, interwoven with the gluteal muscles and the iliotibial band. By integrating insights from quadruped comparisons, Dr. Eng’s groundbreaking research, and modern movement demands, practitioners can more effectively diagnose, treat, and prevent TFL-related pathologies. In doing so, we honor the evolutionary journey that shaped this small but mighty muscle and continue unlocking its potential to support the resilient, upright movement that defines our species.
References
- Eng, C. M., Lieberman, D. E., Biewener, A. A., & Vinyard, C. J. (2015).
The human iliotibial band is specialized for elastic energy storage compared with the chimp fascia lata.
Journal of Experimental Biology, 218, 2382–2390.
https://doi.org/10.1242/jeb.121160 - Eng, C. M., Lieberman, D. E., Biewener, A. A., & Vinyard, C. J. (2015).
The capacity of the human iliotibial band to store elastic energy during running.
Journal of Biomechanics, 48(14), 3847–3854.
https://doi.org/10.1016/j.jbiomech.2015.09.030 - Eng, C. M. (2015). Understanding the IT band.
Harvard Gazette.
https://news.harvard.edu/gazette/story/2015/xx-xx-xx/
(Exact publication date and URL may vary; use the correct link from the Gazette archives.) - Lieberman, D. E. (2011).
The Evolution of the Human Head.
Cambridge, MA: Harvard University Press. - Bramble, D. M., & Lieberman, D. E. (2004).
Endurance running and the evolution of Homo.
Nature, 432(7015), 345–352.
https://doi.org/10.1038/nature03052 - Dyce, K. M., Sack, W. O., & Wensing, C. J. G. (2010).
Textbook of Veterinary Anatomy (4th ed.).
St. Louis, MO: Saunders/Elsevier.
(Contains comparative anatomy references for quadruped muscular and fascial structures.) - Neumann, D. A. (2016).
Kinesiology of the Musculoskeletal System: Foundations for Rehabilitation (3rd ed.).
St. Louis, MO: Elsevier.
(Discusses TFL, hip stabilizers, and the biomechanics of gait.)
Notes:
- Eng et al. (2015): Directly underpins the paragraphs about the IT band’s elastic function and chimp vs. human comparisons.
- Lieberman et al. references: Provide broader evolutionary context for bipedal locomotion, endurance running, and morphological adaptations.
- Dyce, Sack, & Wensing (2010): Standard text for veterinary anatomy, helpful for understanding TFL-like structures in quadrupeds.
- Neumann (2016): Commonly used in rehabilitation and clinical settings for deeper insights into the TFL’s role and interplay with the IT band.