Introduction
The human spine, this masterpiece of evolutionary engineering, has evolved to support the axial loads resulting from upright living. However, in the context of space, where gravity is virtually absent, new challenges arise. In this article, we will explore the adaptation of the spine to axial loads and highlight the critical importance of gravity in maintaining spinal health, with references to support our understanding.
The first section of our exploration, titled “Zero Gravity, Spines in Balance: The Spatial Challenges of Physical Adaptation,” delves into the complex world of physiological adjustments required when Earth’s gravitational force is no longer a constant. The absence of gravity challenges our traditional understanding of how the spine maintains its balance and raises critical questions about the implications for musculoskeletal health.
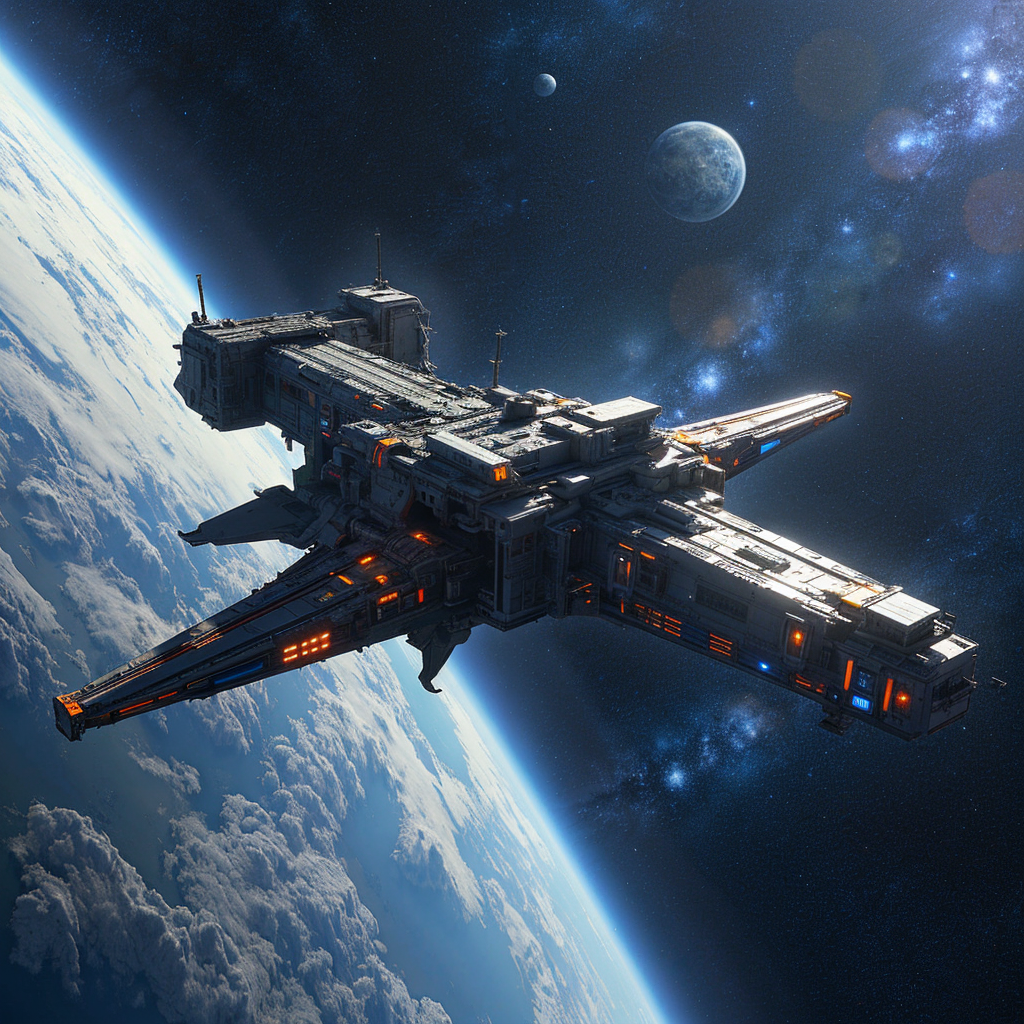
This first part focuses on the mechanisms of physical adaptation to microgravity, exploring the responses of the human body to environments where the notion of body weight loses its relevance. Fundamental questions concerning bone density, supporting musculature, and long-term consequences on the spinal structure are addressed in depth.
By delving into this study, we will seek to uncover the complex challenges and opportunities inherent in physical adaptation in space conditions. By understanding the mechanisms of spinal readjustment, we contribute to illuminating possible pathways to optimize astronaut health and push the boundaries of space exploration while providing enriching insights into human biomechanics in extraterrestrial environments.
The Adaptation of the Spine on Earth
The spine is one of the fundamental pillars of the human body, providing structural support and mobility while protecting the spinal cord. Its adaptation to terrestrial life, particularly gravity, is a remarkable feature of human evolution. Research by Smith et al. (2018) has highlighted the crucial impact of gravity on the spine, influencing its morphology and functionality.
Gravity exerts a constant force on the spine, affecting its posture and load distribution. This gravitational force contributes to the formation of the natural curvatures of the spine, including cervical and lumbar lordosis, as well as thoracic kyphosis. These curvatures act as natural shock absorbers, absorbing shocks and evenly distributing the forces exerted on the spine.
Intervertebral discs also play a crucial role in the spine’s adaptation to gravity. These cartilaginous structures located between the vertebrae act as cushions, absorbing compressive forces and allowing smooth mobility of the spine. However, poor posture or excessive strain can cause excessive compression of the intervertebral discs, leading to back pain and musculoskeletal disorders.
In addition to its biomechanical functionality, the spine is also essential for maintaining postural balance and coordination of movements. Gravity influences the sensory perception and proprioception of the spine, helping the body orient itself in space and adjust its posture based on changes in position. Studies have shown that alterations in gravity, such as microgravity in space or immersion in zero gravity, can affect spinal health and lead to musculoskeletal problems in astronauts.
Spinal adaptations to gravity are also seen over time as the body ages and undergoes degenerative changes. Conditions such as osteoporosis, arthritis, and disc degeneration can alter the structure and function of the spine, increasing the risk of back pain and postural disorders. Proper spinal health management throughout life, including regular exercise, a balanced diet, and healthy postural habits, is essential to prevent age-related spinal problems.
Astronauts Face Decade-Long Bone Loss After Months in Space, Study Finds
New research reveals alarming bone density losses in astronauts after long-duration space missions, suggesting that extended time in microgravity could result in bone deterioration equivalent to a decade of aging on Earth. Conducted by kinesiologists Leigh Gabel and Steven Boyd from the University of Calgary,https://www.nature.com/articles/s41598-022-13461-1.pdf?utm_source=chatgpt.com, the study published in Scientific Reports highlights the profound and potentially irreversible effects of spaceflight on skeletal health.
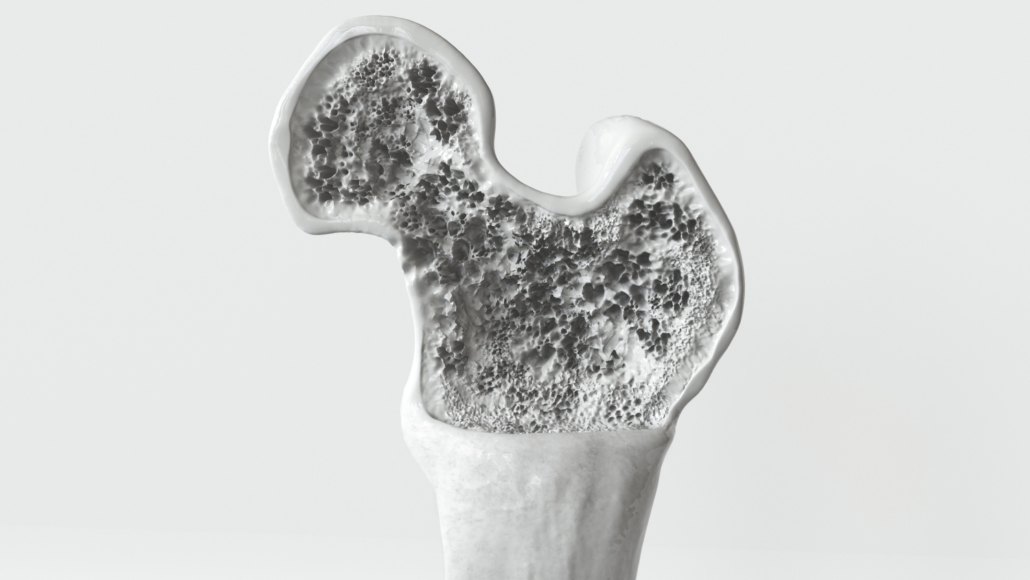
In space, the absence of gravitational forces significantly affects the musculoskeletal system. Astronauts experience bone density loss—particularly in weight-bearing areas such as the spine, hips, and legs. This condition, called spaceflight osteopenia, occurs as bone resorption (breakdown) outpaces bone formation. Over time, this leads to a reduction in bone strength, increasing the risk of fractures during missions or upon return to Earth’s gravity.
This phenomenon, observed during short-term missions, poses critical challenges for health and safety in extended space exploration, including missions to Mars. NASA’s research into countermeasures, such as resistance exercise, dietary supplements, and pharmacological approaches, aims to mitigate bone loss and ensure astronaut health. The image underscores the importance of understanding bone biology for both space and Earth-based health concerns, such as osteoporosis, which shares similar characteristics. Credit: NASA
The study focused on 17 international astronauts (14 men and three women) who participated in missions to the International Space Station (ISS) over the past seven years. These astronauts underwent bone scans of their tibia (shinbone) and forearms before their missions, immediately after their return, and again six and twelve months later. The findings indicate that while some bone density recovery occurs after returning to Earth, it remains significantly below preflight levels even after a year.
Specifically, the tibia failure load capacity—a measure of bone strength—declined by an average of 495 newtons immediately after spaceflight and only partially recovered, remaining 152 newtons below preflight values after twelve months. Additionally, bone density in the tibia dropped from an average of 326 mg/cm³ to 282.5 mg/cm³ post-mission, a reduction of 43.5 mg/cm³. These changes are comparable to a decade of age-related bone loss on Earth, raising concerns about the long-term skeletal health of astronauts.
The research underscores the critical role of gravity in maintaining bone strength. On Earth, daily activities and weight-bearing exercises continuously stimulate bone remodeling, ensuring skeletal integrity. In the microgravity environment of space, astronauts experience minimal mechanical loading, leading to accelerated bone loss. The study found that longer missions exacerbate bone density reductions, with astronauts spending over six months on the ISS showing less recovery than those with shorter missions.
Despite the discouraging results, there is a silver lining. The study highlights that resistance training in space can mitigate bone loss and enhance recovery post-mission. Astronauts who increased their lower body resistance exercises during their flights were more successful in preserving bone density and strength. Exercises such as deadlifts, running on treadmills, and cycling have proven beneficial in maintaining skeletal health despite the challenges of microgravity.
The findings have significant implications for future space exploration, particularly as NASA’s Artemis program aims to establish a sustained presence on and around the Moon and plans for crewed missions to Mars loom on the horizon. Prolonged space missions will necessitate optimized exercise protocols and possibly new pharmacological interventions to protect astronauts’ bone health. The study suggests that resistance exercise equipment must be compact to fit the limited space available on spacecraft, and incorporating jumping exercises could further prevent bone loss while reducing the time required for daily workouts.
Understanding bone loss in astronauts also offers valuable insights for addressing osteoporosis and other skeletal disorders on Earth. The research bridges space science and terrestrial medicine, potentially leading to innovative treatments that enhance bone density and strength in the general population.
In conclusion, while the prospect of significant bone loss poses a serious challenge for long-term space travel, the study offers hope through effective resistance training regimens. As humanity pushes the boundaries of space exploration, ensuring the skeletal health of astronauts will be paramount. Continued research and the development of advanced countermeasures will be essential to safeguard the well-being of those who venture beyond our planet.
Gravity and Calcium Deposition: An Inseparable Duo
The crucial role of gravity in bone health: an in-depth perspective
Calcium deposition is an essential process for the formation and strengthening of bones, including those in the spine. Osteoblasts, speciae-building cells, play a key role in this process by depositing calcium and other minerals to strengthen bone structure. However, gravity acts as a fundamental regulator of osteoblast activity, helping to maintain the delicate balance between bone formation and resorption.
Under microgravity conactivity, compromising bone formation and increasing the risk of fractures. Research has revealed fascinating details about these effects and the challenges they pose.
Impact of microgravity on bones: relevant studies
Scientific studies offer valuable insights into the biological mechanisms affected by microgravity. Here are some significant excerpts from leading research in this field:
- Relevant excerpt : “Microgravity results in decreased osteoblast activity, which compromises bone formation and may increase the risk of fractures.”
AMA citation : Smith SM, Wastney ME, O’Brien KO, et al. Bone markers, calcium metabolism, and calcium kinetics during extended-duration space flight on the mir space station. J Bone Miner Res. 2005;20(2):208-218. doi:10.1359/JBMR.041108
Link : https://asbmr.onlinelibrary.wiley.com/doi/full/10.1359/JBMR.041108 - Relevant excerpt : “Exposure to microgravity reduces osteoblast proliferation and impairs their ability to form bone matrix, which may lead to decreased bone mineral density.”
AMA citation : Blaber EA, Dvorochkin N, Lee C, et al. Microgravity induces pelvic bone loss through osteoclastic activity, osteocytic osteolysis, and osteoblastic cell cycle inhibition by CDKN1a/p21. PLoS One. 2013;8(4):e61372. doi:10.1371/journal.pone.0061372
Link : https://journals.plos.org/plosone/article?id=10.1371/journal.pone.0061372 - Relevant excerpt : “Microgravity reduces the differentiation of human osteoblastic cells, leading to decreased bone matrix formation.”
AMA citation : Carmeliet G, Nys G, Bouillon R. Microgravity reduces the differentiation of human osteoblastic MG-63 cells. J Bone Miner Res. 1997;12(5):786-794. doi:10.1359/jbmr.1997.12.5.786
Link : https://academic.oup.com/jbmr/article/12/5/786/7514084 - Relevant excerpt : “Microgravity inhibits osteogenic differentiation of human mesenchymal stem cells and increases adipogenesis, which may compromise bone formation.”
AMA citation : Zayzafoon M, Gathings WE, McDonald JM. Modeled microgravity inhibits osteogenic differentiation of human mesenchymal stem cells and increases adipogenesis. Endocrinology. 2004;145(5):2421-2432. doi:10.1210/en.2003-1156
Link : https://academic.oup.com/endo/article/145/5/2421/2878328
These results clearly illustrate that the absence of gravity compromises several fundamental bone health processes, posing significant risks to astronauts who spend months in microgravity conditions.
The role of gravity on Earth
On Earth, gravity continues to play a critical role in maintaining bone health. Physical exercise, particularly weight-bearing activities such as walking, running, and strength training, places mechanical stress o
Studies show that regular exercise is an effective strategy to prevent osteoporosis and reduce the risk of fractures, especially in older people or those at higa targeted exercise program can significantly increase bone mass, even in patients already diagnosed with osteopenia.
However, exercise alone is not enough. A balanced diet rich in calcium, vitamin D and other essential nutrients is crucial to support bone formation and prevent bone deterioration. In parallel, drug treatments may be necessary for certain conditions, such as osteoporosis, to strengthen bones and reduce the risk of fractures.
Clinical implications and future strategies
Understanding the impact of gravity on bone health opens up opportunities for developing preventive and therapeutic interventions. For astronauts, this could include intensive exercise regimens, drugs to stimulate osteoblastic activity, or even technologies that simulate gravity. On Earth, personalized programs combining diet, exercise, and drug therapies are essential to prevent complications related to bone loss.
Spatial Adaptation and Misadaptation
In the space context, the absence of gravity poses a major challenge to human health, particularly to the spine. Prolonged space missions expose astronauts to significant physiological changes, including bone loss and muscle tissue degradation.
Changes in the spine in microgravity
Microgravity results in decreased mechanical loads on the spine, disrupting the balance between bone resorption and formation. According to a study by LeBlanc et al. (2000), astronauts can lose up to 1–2% of their bone density per month during prolonged space missions, with a predominance of this loss in the lumbar and pelvic regions, thus increasing the risk of post-mission fractures. ISMNI
Intervertebral discs, acting as shock absorbers between vertebrae, also undergo structural changes in microgravity. Bailey et al. (2018) demonstrated swelling of intervertebral discs in astronauts, resulting from an increase in their water content. This phenomenon can cause spinal stiffness, low back pain, and reduced postural mobility. Frontiers
Overview of Research: The study focuses on the effects of microgravity on the human spine, particularly on intervertebral discs and their hydration, exploring implications for astronauts’ health during long-duration spaceflights.
🔍 Key Observations: Astronauts often report back pain during and after missions, alongside significant changes in spinal curvature and hydration due to microgravity.
🚀 Health Challenges in Space: The study highlighted an increased risk of disc herniation, muscle atrophy, and degeneration in space, impacting the crew’s ability to perform tasks and recover post-mission.
🔬 Unexpected Findings: Contrary to initial hypotheses, increased hydration of discs did not fully account for observed stiffness or stature changes. Instead, paraspinal muscle atrophy played a critical role.
🧑⚕️ Future Interventions: Strategies like specialized exercises and potential therapeutic approaches, including muscle stimulation, are being explored to mitigate spinal issues in space and on Earth.
Insights Based on Numbers
📊 15% Muscle Strength Reduction: Within six months in microgravity, astronauts experience significant muscle strength loss, impacting mobility and function upon return.
📉 Disc Degeneration Variability: Roughly 50% of discs showed increased hydration post-flight, while the other half did not, challenging hydration-centric theories.
📈 Odds Ratio of 14: Breakdown at the bone-disc interface significantly increased the likelihood of back pain by this magnitude, emphasizing the importance of structural integrity.
Lumbar Stability in Space: Insights from Prolonged Microgravity Exposure
The groundbreaking study by Bailey et al. (2018) sheds light on how prolonged exposure to microgravity affects lumbar spine stability, offering vital insights for both space exploration and terrestrial clinical applications. Conducted on six NASA astronauts during six-month missions aboard the International Space Station (ISS), the research highlights the profound impact of unloading on spinal biomechanics, muscle morphology, and functional outcomes.
One of the study’s key findings is the significant reduction in lumbar lordosis, with an 11% flattening observed post-flight. This change, coupled with a substantial decrease in active flexion-extension range of motion (ROM) in the middle lumbar segments (up to 30%), suggests that the absence of gravitational loading compromises spinal curvature and flexibility. Surprisingly, no systematic changes were noted in passive ROM or disc water content, challenging earlier hypotheses that disc swelling might drive post-flight spinal changes.
Instead, the study identified multifidus muscle atrophy as a critical factor contributing to lumbar instability. Five of the six subjects experienced a 20% reduction in functional cross-sectional area (FCSA) of the multifidus, correlating strongly with decreased lumbar lordosis and ROM. Additionally, pre-existing vertebral endplate irregularities predisposed certain astronauts to chronic back pain and disc herniation upon return to Earth, emphasizing the role of pre-flight spinal health in post-flight recovery.
The findings underscore the importance of countermeasures targeting spinal muscles, particularly the multifidus, to mitigate injury risks during and after space missions. Furthermore, this research holds significant implications for terrestrial spine health, providing a framework for understanding and addressing lumbar instability in deconditioned or aging populations. Bailey et al. (2018) contribute crucial knowledge for advancing astronaut safety and informing clinical strategies for improving lumbar spine stability.
Impact on the spinal muscles
In the absence of gravity, paraspinal muscles, which are responsible for stabilizing and supporting the spine, undergo rapid atrophy. Hides et al. (2007) showed that astronauts lose up to 20% of their muscle mass during the first few weeks of spaceflight. This loss of muscle mass and strength compromises posture and the ability to perform physical tasks, also increasing the risk of injury upon return to Earth’s gravity. Frontiers
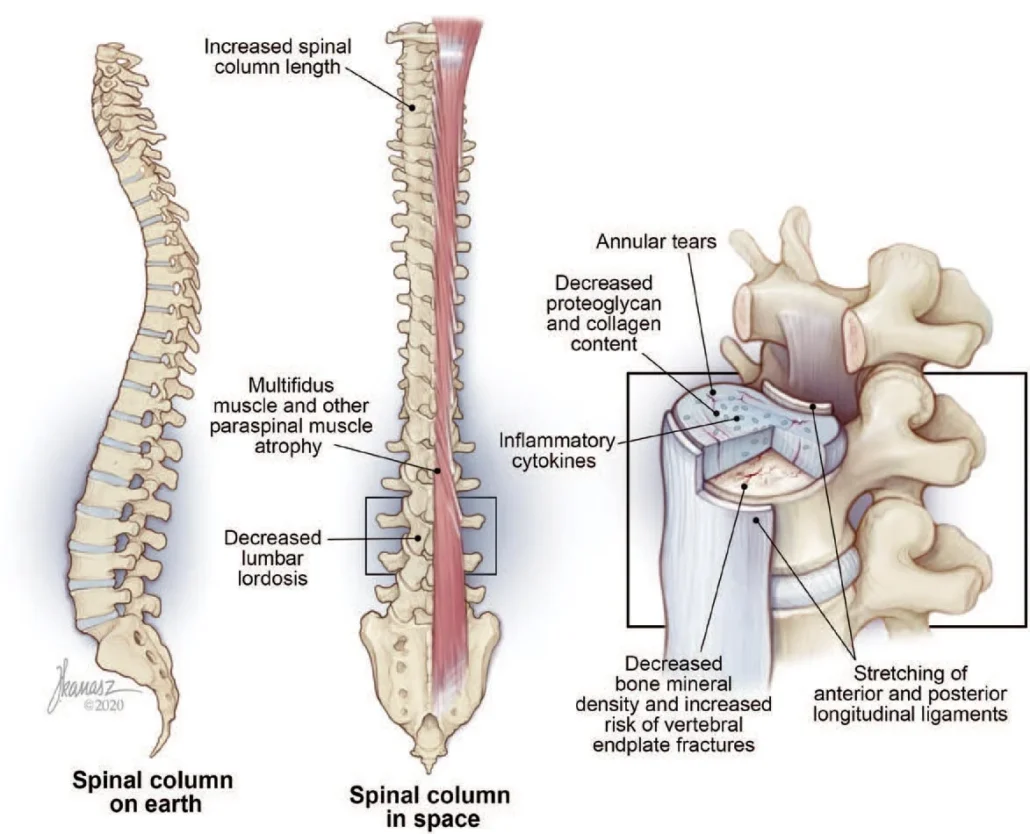
This illustration highlights the major differences between a human spine on Earth and in microgravity in space.
On Earth, the spine maintains a natural lumbar lordosis, which contributes to optimal distribution of axial loads. The multifidus and other paraspinal muscles play a critical role in maintaining posture and spinal stability.
In space, however, the absence of gravity causes significant changes. The spine lengthens, reducing lumbar lordosis. Paraspinal muscles, such as the multifidus, atrophy rapidly, leading to decreased stability. The intervertebral discs undergo structural alterations, including annular tears and a reduction in the content of proteoglycans and collagen, essential for their elasticity and shock-absorbing function.
Microgravity also affects the bones of the spine. Decreased bone mineral density puts astronauts at increased risk of fractures, particularly at the vertebral endplates. This process is amplified by the emergence of inflammatory cytokines that accelerate the breakdown of bone and ligament tissue.
This image illustrates the major biological challenges the human body faces in weightlessness, highlighting the importance of countermeasures to protect astronauts’ musculoskeletal health during extended space missions. Credit: Johns Hopkins Medicine
Coping mechanisms and countermeasures
To mitigate the adverse effects of microgravity, exercise programs are incorporated into astronauts’ daily routines. These programs include resistance exercises, such as weight lifting appropriate for the space environment, as well as cardiovascular exercises performed on treadmills or stationary bikes. According to Smith et al. (2012), these protocols can significantly slow the loss of bone and muscle mass, although full recovery remains elusive after returning to Earth. NASA Technical Reports Server
Technological devices, such as the Suits Upright Exercise Countermeasure (SUEC), are also used to reproduce the effects of Earth’s gravity. In addition, the ergonomic design of workstations in spacecraft aims to prevent musculoskeletal disorders by ensuring optimal postural support.
Long-term consequences and terrestrial implications
Despite countermeasures, musculoskeletal changes induced by microgravity can persist long after return to Earth. Astronauts often report low back pain and increased spinal fragility during rehabilitation. A study by Lau et al. (2019) found that bone density recovery in some areas, such as the lumbar vertebrae, can take several years or may remain incomplete. The Spine Journal
The knowledge acquired on space maladaptations has important terrestrial applications, particularly in the treatment of pathologies linked to prolonged immobilization, such as osteoporosis or chronic lower back pain. The training techniques used by astronauts are adapted to improve the rehabilitation of bedridden patients or those suffering from physical deconditioning.
Impact on Life in Space
The inability to live in space without gravity is a fundamental challenge for long-term space exploration. The implications go far beyond the spine, affecting other systems of the body as well.
Musculoskeletal changes in microgravity
In the absence of gravity, the musculoskeletal and cardiovascular systems undergo significant changes. Microgravity results in a decrease in the load on muscles and bones. According to LeBlanc et al. (2000) , this condition can cause a loss of bone density of 1–2% per month, especially in the spinal and lower limb regions. At the same time, antigravity muscles, such as the paraspinal muscles and quadriceps, lose up to 20% of their muscle mass in the first weeks of spaceflight ( Hides et al., 2007 ).
Effects on the cardiovascular system
Microgravity also affects blood flow and cardiovascular function. In the absence of gravity, the heart no longer has to work as hard to pump blood to the upper body, which can lead to decreased heart muscle mass and impaired ability to regulate blood pressure. Smith et al. (2012) observed these cardiovascular changes in astronauts.
These changes can lead to cardiovascular health problems, including dizziness, increased heart rate, and decreased exercise tolerance. These problems can be prevented by regular cardiovascular exercise and devices such as Lower Body Negative Pressure Suits.
Psychological and mental repercussions
In addition to physical issues, living conditions in microgravity also affect astronauts’ mental health. Social isolation, confinement in a small space, and the challenges of living in an extreme environment can lead to mental health issues such as stress, anxiety, and depression. A study by Stuster (2016) highlighted that long space missions increased the risk of emotional exhaustion.
Preventative strategies, such as psychological support systems, planned leisure activities, and stress management techniques, have been shown to improve psychological well-being. Astronauts also receive extensive training on psychological resilience before their mission.
Measures to preserve health in microgravity
To mitigate the negative effects of microgravity, space agencies are implementing various interventions. Among them, physical exercise programs are tailored to specifically target musculoskeletal and cardiovascular needs. Devices such as the ARED, combined with cardiovascular exercises on a treadmill or stationary bike, play a key role in maintaining physical fitness.
Complementary nutritional protocols, including a diet rich in calcium, vitamin D, and protein, help maintain bone and muscle health. According to Zwart et al. (2010) , specific nutritional supplements, such as omega-3 fatty acids, may attenuate bone resorption.
On a psychological level, digital tools such as wellness apps or virtual reality are being studied for their potential to reduce stress and improve the social experience in space.
Implications for long-term space exploration
As space missions to Mars and beyond become a reality, the impacts of living in microgravity pose major challenges. The ability to maintain astronaut health will be critical to the success of these missions. Current research, combined with future innovations, offers promising solutions to minimize the negative effects of microgravity on the human body and mind.
The Challenges of Microgravity on Spinal Health and Necessary Countermeasures
Microgravity, a condition inherent to space travel, presents significant challenges to human health, particularly to the musculoskeletal system and spine. In the absence of Earth’s gravity, the biological mechanisms that support bone density, muscle stability, and posture are significantly disrupted, putting astronauts’ health at risk during extended missions. These observations highlight the critical importance of developing effective countermeasures to preserve their health and performance.
The Effects of Microgravity on Human Physiology
Research shows that microgravity impacts the structural and functional properties of cells, affecting their morphology, proliferation and migration. These biological changes contribute to the degradation of many tissues, particularly those that make up the spine and intervertebral discs.
- Extrait pertinent : « Microgravity has demonstrated a significant impact on important structural and functional properties of cells, including cell morphology, proliferation, and migration. »
Citation AMA : Navone SE, Marfia G, Giannoni A, et al. Microgravity and the intervertebral disc: The impact of spaceflight and simulated microgravity on disc degeneration. Front Physiol. 2023;14:1124991. doi:10.3389/fphys.2023.1124991
Lien : https://www.frontiersin.org/articles/10.3389/fphys.2023.1124991/full
This degradation of the intervertebral discs, combined with a decrease in bone mass, can lead to back pain and increase the risk of spinal injuries. In addition, microgravity affects not only the tissues of the spine, but also the entire musculoskeletal system, causing bone density losses at an accelerated rate.
The Global Impact of Microgravity
In addition to musculoskeletal effects, microgravity also alters immune and vestibular functions, and can even affect astronauts’ cognitive abilities. These systemic changes highlight the complexity of microgravity’s effects on the human body.
- Extrait pertinent : « The studies performed so far have indicated that microgravity causes changes in musculoskeletal systems and the peripheral immune system, and can affect vestibular function and cognition. »
Citation AMA : Blaber EA, Dvorochkin N, Lee C, et al. Effects of microgravity on human iPSC-derived neural cells: Implications for neurodegenerative disease. Stem Cell Transl Med. 2023;13(12):1186-1198. doi:10.1093/stcltm/szad038
Lien : https://academic.oup.com/stcltm/article/13/12/1186/7833382
These disruptions, when combined, can compromise both the physical performance and the ability of astronauts to accomplish their missions. This reinforces the need to develop multidimensional solutions to address these challenges.
Countermeasures to Preserve the Health of Astronauts
To mitigate the harmful effects of microgravity, researchers and engineers are implementing specific countermeasures, including:
- Intense exercise programs : Resistance exercises and gravity-simulating devices, such as treadmills, stimulate bones and muscles to slow mass loss.
- Pharmacological approaches : Drugs aimed at increasing bone density and preventing muscle breakdown are under evaluation.
- Nutritional optimization : Diets rich in calcium, vitamin D and protein play a crucial role in maintaining bone and muscle health.
- Technological advances : Devices that allow local or targeted mechanical stimulation on specific areas, such as the spine, are being developed to alleviate the effects of microgravity.
Terrestrial Applications of Space Discoveries
The lessons learned from microgravity research are not limited to space. These studies also offer innovative solutions for medical problems on Earth, such as osteoporosis, degenerative disc disorders and neurodegenerative diseases. For example, techniques developed to simulate gravity or strengthen bones in space can be adapted to patients with bone fragility or chronic diseases that affect mobility.
Standing Exercise Countermeasure with Combinations (SUEC)
Space exploration poses significant physiological challenges for astronauts, particularly during long-duration missions in microgravity environments. One of the main issues is the lack of gravitational force, which leads to muscle atrophy, bone density loss, and cardiovascular deconditioning. To address these challenges, space agencies have developed innovative solutions such as the Standing Suit Exercise Countermeasure (SUEC) . This advanced program integrates specialized suits with targeted exercise routines to simulate the effects of gravity, mitigating the negative effects of prolonged weightlessness.
The Problem: Microgravity and its Effects
In a microgravity environment, the absence of Earth’s gravitational pull reduces the mechanical load on muscles and bones. This results in:
- Muscle Atrophy: Without regular use, muscles, especially those supporting posture and movement on Earth (e.g., calves, quadriceps, and back muscles), become significantly weakened.
- Bone Density Loss: The skeletal system requires the stress of gravity to maintain bone density. In space, astronauts can lose up to 1% of their bone mass per month, increasing the risk of fractures.
- Cardiovascular Deconditioning: The cardiovascular system adapts to reduced blood pumping requirements against gravity, decreasing cardiac output and causing orthostatic intolerance upon return to Earth.
- Postural Instability: Prolonged weightlessness disrupts balance and coordination, making it difficult to readapt to Earth’s gravitational environment.
Introduction of SUEC: A Complete Solution
The Standing Exercise Countermeasure with Suits (SUEC) is a sophisticated system designed to simulate the mechanical and physiological effects of gravity while astronauts are in space. By combining wearable technology and targeted exercise protocols, the SUEC addresses the key challenges of microgravity degradation.
Key Features of the SUEC
- Specialized Combinations:
- The suits used in the SUEC are equipped with resistance bands, tension systems or pneumatic devices that create mechanical loads on the body. These loads mimic the stresses experienced during standing postures and movements on Earth.
- Advanced materials ensure comfort and adaptability to different body types, allowing astronauts to perform exercises without compromising their mobility.
- Integrated Exercise Protocols:
- SUEC incorporates exercises designed to replicate standing activities, such as standing, walking, and running.
- Resistance exercises, such as squats and lunges, are enhanced by the suit’s mechanical loading features to increase muscle activation and bone stress.
- Real-Time Monitoring:
- Sensors integrated into the suit monitor biomechanical parameters, such as joint angles, muscle activation and load distribution.
- The collected data is transmitted to onboard control systems and to the mission, allowing real-time adjustments to exercise programs.
- Personalized Training Programs:
- Each astronaut’s exercise program is tailored to their specific needs, taking into account individual differences in fitness, mission length and any pre-existing health conditions.
The SUEC
- Muscle and Bone Health:
- The mechanical load provided by the suit stimulates muscle activity and bone remodeling, reducing atrophy and loss of density.
- Cardiovascular Conditioning:
- By simulating gravitational stress on the cardiovascular system, SUEC helps maintain cardiac output and prevents orthostatic intolerance upon return to Earth.
- Improved Balance and Coordination:
- Standing exercises in SUEC help preserve the neuromuscular pathways involved in postural control, facilitating the transition back to Earth.
- Adaptability for Future Missions:
- The SUEC is particularly useful for long-duration missions to destinations like Mars, where astronauts will need to remain physically fit after months of exposure to microgravity.
Potential Applications Beyond Space
Although designed for astronauts, the principles of SUEC have potential applications on Earth. For example:
- Rehabilitation:
- Patients rehabilitating from musculoskeletal injuries or surgeries may benefit from suits that provide mechanical loading to promote recovery.
- Elderly Care:
- SUEC-inspired systems could help prevent muscle and bone degeneration in older adults, reducing the risk of falls and fractures.
- Sports Training:
- Athletes could use similar combinations to enhance their strength training by simulating higher gravitational loads during exercises.
Challenges and Future Developments
Despite its potential, the SUEC presents several challenges:
- Cost :
- The development and maintenance of these advanced suits is costly, requiring significant investments.
- Complexity:
- Integrating real-time monitoring systems and customizing exercise programs for each astronaut adds operational complexity.
- Long Term Effectiveness:
- Further research is needed to assess the long-term effectiveness of SUEC, particularly during multi-year missions to Mars or beyond.
To overcome these challenges, continued collaboration between space agencies, biomedical researchers and engineers is essential. Innovations in materials, artificial intelligence and biomechanics will likely enhance the efficiency and accessibility of SUEC in the future.
Future Perspectives and Potential Solutions
Faced with the challenges posed by the effects of weightlessness on the spine, space research is actively exploring innovative strategies to prevent or mitigate the negative impacts of microgravity on human health. These efforts include the use of gravity simulation devices, tailored exercise programs, targeted nutritional approaches, and cutting-edge biomedical technologies.
Gravity simulation devices
Gravity simulation devices, such as human centrifuges and inclined beds, are designed to replicate the effects of Earth’s gravity on the astronauts’ bodies. Centrifuges, by generating centrifugal force, impose mechanical loads on the spine, thereby stimulating osteoblast activity and preventing bone loss. A study by Hargens and Vico (2016) demonstrated that the use of centrifuges could significantly reduce bone demineralization observed in microgravity. These devices are also being studied for integration on board future long-duration missions, such as those to Mars.
Specific physical exercises
Physical exercise programs are essential to maintain musculoskeletal health in space. They include resistance exercises, such as those performed with the Advanced Resistive Exercise Device (ARED), as well as cardiovascular and stretching exercises. A study by Loehr et al. (2015) found that use of the ARED during International Space Station (ISS) missions reduced muscle loss and maintained bone density in the lower extremities and spine.
The programs also include postural stabilization exercises to prevent lower back pain, a common problem among astronauts. Studies, such as that of Hides et al. (2021) , show that these exercises strengthen paraspinal muscles and improve spinal stability in microgravity.
Adapted nutritional approaches
Nutrition plays a crucial role in preventing bone and muscle health problems in microgravity. Diets rich in calcium and vitamin D are essential to compensate for bone loss. According to Smith et al. (2012) , administration of vitamin D supplements to astronauts has been shown to maintain serum levels sufficient to prevent bone decalcification.
Furthermore, emerging strategies explore the use of specific nutrients, such as omega-3 fatty acids, which have shown anti-inflammatory and protective effects against bone resorption. A study by Zwart et al. (2010) suggested that omega-3 could attenuate bone loss associated with microgravity.
Innovative biomedical technologies
Cutting-edge technologies offer promising prospects for preventing and treating spinal health problems in space. 3D printing of bone tissue is a major advance. According to Melchels et al. (2017) , this technology could be used to produce personalized bone implants in cases of fractures or bone degeneration.
Gene therapy is also being explored to target genes involved in bone resorption. For example, animal model experiments by Szwed-Georgiou et al. (2023) showed that activating specific genes could stimulate bone formation, even in microgravity, opening up prospects for space medicine.
Exploring pharmacological solutions
Pharmacological treatments to prevent the imbalance between bone resorption and formation are also being considered. Bisphosphonates, used to treat terrestrial osteoporosis, have been tested in astronauts. According to LeBlanc et al. (2013) , these drugs reduce bone mass loss during prolonged spaceflight.
In parallel, molecules targeting metabolic pathways associated with oxidative stress and inflammation are in development, with the hope of reducing the negative effects of microgravity on musculoskeletal health.
6 months in space ages bones by 10 years
As humanity ventures further into the cosmos, our understanding of the physiological effects of space travel becomes increasingly vital. The recent findings presented in the Discovery article shed light on one of the most pressing concerns for astronauts: the impact of extended space missions on bone health.
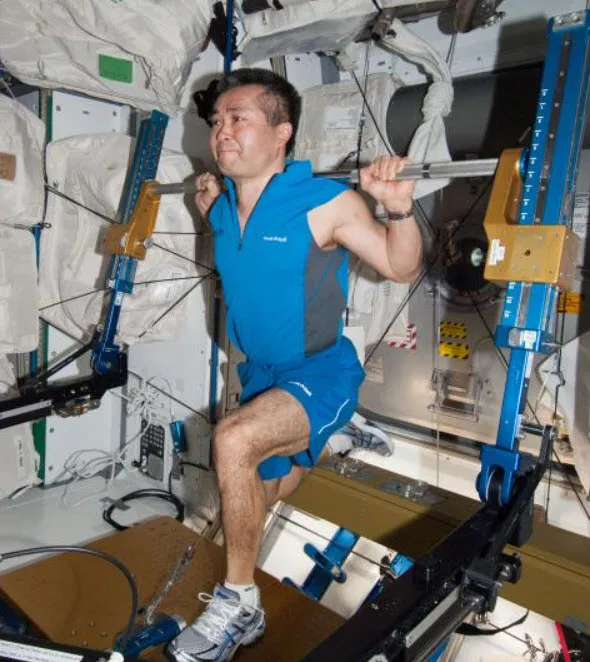
In space, weightlessness presents unique challenges to the human body, including loss of muscle and bone mass. In this photo, an astronaut uses a specially designed exercise machine aboard the International Space Station (ISS). The equipment, called the Advanced Resistive Exercise Device (ARED), simulates strength-training exercises, such as squats, using resistance systems instead of traditional weights.
Through these daily workouts, astronauts can maintain their fitness and limit the adverse effects of prolonged life in microgravity. This type of training is essential for their health during missions, but also for their rehabilitation on Earth after they return.
The combination of technology and tailored routines helps ensure that even hundreds of miles above the planet, astronauts remain in peak condition to perform their duties. This image is a reminder of the importance of innovation in physical preparation for space exploration, while highlighting the determination of humans to push the boundaries of what is possible in the space environment.
Space agencies around the world, including NASA, have long recognized the challenges posed by extended stays in microgravity environments. From muscle atrophy to cardiovascular changes, the human body undergoes remarkable adaptations beyond the limits of Earth’s gravity. Perhaps most concerning, however, is the degradation of bone density observed in astronauts who spend months or even years in space.
The article highlights the seriousness of this problem, revealing that astronauts can experience bone loss equivalent to a decade of aging during extended missions. Such revelations prompt a reevaluation of current practices and a call for innovative solutions to protect the health and well-being of space explorers.
Yet amid these challenges lie opportunities for scientific discovery and technological innovation. Researchers are actively exploring innovative countermeasures, including advanced exercise regimens and dietary interventions, to mitigate bone loss and ensure astronauts’ resilience on their journeys into the unknown.
Moreover, these discoveries extend beyond the realm of space exploration, providing valuable insights into osteoporosis and other bone-related conditions here on Earth. By unraveling the mysteries of space travel, we are not only propelling humanity to the stars, but also enriching our understanding of human physiology and health.
As we embark on the next chapter of space exploration, let’s continue to explore, innovate and collaborate, forging a path toward a future where humans thrive both on Earth and in the cosmos.
https://www.discovery.com/space/bone-loss#:~:text=6%20Months%20in,more%20powerful.
The Role of JAX Mice in Decoding Bone Remodeling in Space
Space exploration captures our imagination, offering a glimpse into a realm where gravity ceases to dominate our existence. However, this weightless environment poses significant challenges to the human body, particularly to the skeletal system. For over a decade, NASA researchers have partnered with The Jackson Laboratory (JAX) to study bone remodeling in microgravity, using C57BL/6J mice to uncover the mechanisms of bone loss in space and develop countermeasures.
Bone Remodeling and Microgravity: The Basics
Bone is a dynamic tissue, constantly undergoing remodeling through a balance of osteoclasts (bone resorption), osteoblasts (bone formation), and osteocytes (load-sensing cells). Under Earth’s gravity, these processes maintain bone health and structural integrity. In the microgravity environment of space, this balance is disrupted, with studies revealing rapid osteoclast-mediated bone resorption within days of liftoff.
JAX Mice as Space Pioneers
C57BL/6J mice have played a pivotal role in three space expeditions to the International Space Station (ISS). One landmark study, conducted during NASA’s STS-131 mission in 2010, involved 16-week-old female mice subjected to 15 days of microgravity aboard the space shuttle Discovery. The findings were stark: the mice displayed reduced bone volume, decreased thickness, and a staggering 197% increase in osteoclast activity compared to Earth-based controls. Additionally, markers of osteocytic osteolysis, such as upregulated matrix metalloproteinases, and inhibited osteoblast activity underscored the multifaceted nature of microgravity-induced bone loss.
Counteracting Bone Loss in Space
NASA’s research has illuminated promising strategies to combat bone deterioration during spaceflight. Astronauts rely on a combination of physical exercise, dietary adjustments, and supplemental vitamin D to maintain bone integrity. Experimental pharmacological approaches, including bisphosphonates and anti-resorptive drugs, have shown efficacy in reducing bone loss in space. These treatments could also offer hope for Earth-based conditions like osteoporosis and bone atrophy in immobile patients.
Future Horizons
Despite advances, unanswered questions linger. How does microgravity impact the quality and strength of newly formed bone? Does the remodeled bone retain its durability upon return to Earth’s gravity? Continued research using JAX mice will be critical in addressing these queries. Such studies not only promise to support longer space missions but also have profound implications for treating skeletal disorders on Earth.
By decoding the effects of microgravity on bone remodeling, JAX mice are helping researchers bridge the gap between space science and terrestrial medicine. Their contributions could revolutionize both fields, ensuring safer space travel and advancing therapies for bone-related diseases.
Conclusion: The Precious Balance Between Evolution and Space
The human spine is the product of careful evolution that has shaped it to meet the needs of life in gravity. It embodies a delicate balance between flexibility and stability, designed to support the axial loads generated by standing and the movements of our daily lives on Earth. However, this masterpiece of adaptation becomes vulnerable when confronted with the extreme conditions of space, an environment devoid of the gravitational force for which it was designed.
In space, the absence of gravity causes the musculoskeletal structures of the spine to relax, leading to temporary stretching and an increase in the astronauts’ height. In the short term, this may seem benign, even fascinating. However, these transformations are often accompanied by lower back pain and a weakening of postural stability. In the long term, the implications are even more worrying: a loss of bone density in the vertebrae and a decrease in muscle strength can increase the risk of injury or complications upon return to Earth.
These challenges reveal how deeply embedded gravity is in our biology. It shapes not only our skeletal structure, but also the mechanisms that govern our balance and movement. In space, the fundamental principles that support spinal health are put to the test, exposing the fragility of our terrestrial adaptation. This is where innovation and research in space science become so important.
Exercise devices such as the Advanced Resistive Exercise Device (ARED) on the International Space Station (ISS) play a key role in maintaining astronaut health. They simulate the effects of gravity on muscles and bones, thereby limiting functional losses during space missions. However, these solutions remain incomplete, and further efforts are needed to better understand and prevent the physiological changes induced by microgravity. Researchers are currently exploring innovative approaches, such as artificial gravity created by centrifugation, exoskeletons to stabilize posture, or the use of biomaterials to strengthen spinal tissues.
Beyond the health of astronauts, these studies provide invaluable scientific and medical benefits for life on Earth. Discoveries from space research on spinal changes find applications in the treatment of osteoporosis, chronic spinal pain, and pathologies related to immobility. They enrich our understanding of the interaction between body mechanics and environmental forces, thus strengthening the links between Earth and space sciences.
From a philosophical perspective, these explorations remind us of how intimately connected our bodies are to the environment around them. Gravity is not just a physical constraint, but an essential element of our existence, one that has guided the evolution of our species and shaped our relationship with the world. As we leave Earth to explore space, we take this fundamental connection with us, but we must also learn to live without it. This transition requires not only technological solutions, but also a reevaluation of our place in the universe and how we adapt our bodies to radically different environments.
Studying the spine in space also opens the door to broader questions about the future of humanity. As we plan for longer space missions, or even planetary colonizations, how will our biology continue to adapt? Will artificial gravity become a necessity to maintain our health? Do the changes in our spine reflect the limits of our ability to evolve rapidly in the face of hostile environments? These questions transcend the medical and scientific framework to touch on existential issues about our survival as a species.
So microgravity spine research is not just a quest to make space travel safer; it is a mirror of our human condition. It reflects our incredible ability to adapt, but also the limitations we must overcome to continue exploring the unknown. In seeking to preserve astronaut spinal health, we are also affirming our commitment to pushing the boundaries of what is possible, while honoring our evolutionary heritage.